MINING FOR ENERGY TRANSITION
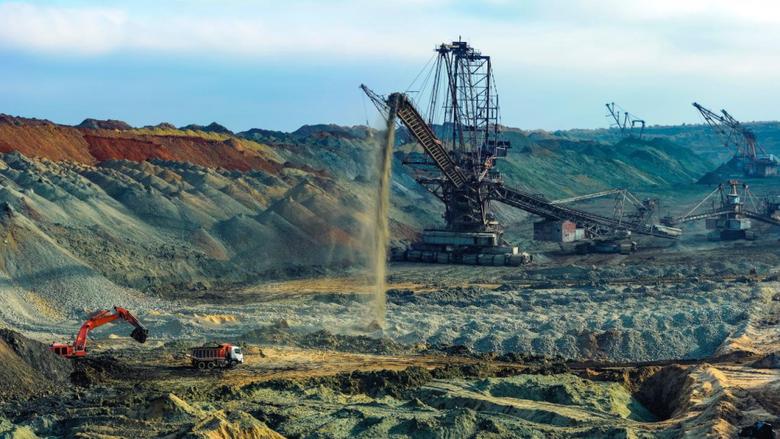
By Ryan McGuine Engineer Alliant Energy
ENERGYCENTRAL - Nov 23, 2020 - There is a profound shift taking place in the way the world produces and consumes energy — from energy sources with high carbon intensities, toward ones with low carbon intensities. The two categories of energy sources differ significantly, the former being one in which energy is embedded in a resource, and the latter being one in which technology is the resource. In order to achieve such a transition fast enough to prevent the worst effects of climate change, a simultaneous reduction of existing fossil fuel assets and buildout of clean energy assets is needed. However, clean energy technologies still depend on natural resource extraction, so the ongoing energy transition is better conceptualized as a shift toward the extraction of different resources, rather than the extraction of fewer.
Today, most of the energy consumed around the world is in the form of either fossil fuels directly, or electricity generated from fossil fuels. But going forward, energy systems will increasingly depend on electricity generated by solar panels and wind turbines, and stored with large batteries. All of those technologies depend on a range of metals and minerals, and demand for them is set to skyrocket — demand for graphite, lithium, and cobalt could increase by 450% by 2050, which would likely raise prices. Indeed, prices for cobalt jumped by 5 times between 2016 and 2018, reflecting temporary supply constraints and rapid demand growth. Supply has since responded to bring the price back down, but such volatility has understandably spurred concern by companies and governments about the short-term supply of these critical metals and minerals.
There are a few important concepts to wrestle with in order to understand the differences in resource paradigms, the first of which is the constantly-shifting economics of natural resources. The world has extracted a lot of natural resources over the last 50 years, but “reserves” of most metals, minerals, and fuels are actually higher today than they were in the 1970s — reserves are merely a working inventory of how much resource is thought to be economically extractable at one moment in time. While the total amount of some resource in the earth cannot change, the depletion of certain reserves, coupled with innovation in both extraction and consumption, causes resources once deemed subeconomic to become economic over time. Thus, while determining which minerals are most at risk in the short term is a valuable task, concern about their long term supply is probably overblown.
The next important concept is energy density. Energy density is the amount of energy per unit mass or per unit volume of some resource. More concentrated sources of energy have important benefits in terms of extraction, transportation, and storage — it explains why it is hard to use batteries to move a container ship, why hydrogen cannot directly replace jet fuel, and why natural gas gets liquified before being transported by sea. Technologies like wind turbines and solar panels are less energy dense than fossil fuels, so they need even more physical materials per unit energy than fossil fuels (as shown below).
Finally, whereas fossil fuels are consumed continuously, minerals are one input of larger manufacturing and infrastructure construction processes. As such, there is less risk from high prices due to supply constraints, but more risk from insufficient manufacturing capacity. In addition, the market structure and supply chains for each are quite different. For example, the mining side of the supply chain is less transparent, and the manufacturing portion is relatively longer, for critical metals and minerals than for fossil fuels. This suggests that the security challenges associated with critical metals and minerals require more complex solutions than merely stockpiling the raw materials.
Clean energy materials involve pollution at both ends of their life cycle. At the beginning, mining creates both local and global pollution. For example, open pit mining generates lots of particulate matter, rocks containing metal ores are often high in sulfides and can acidify water and soil near mines when exposed, the process of separating rare earth elements from the surrounding soil requires hazardous chemicals, and brine mining can damage water quality and reduce water levels. Additionally, mining accounts for around 4-7% of global carbon emissions, since most of the energy it consumes comes from fossil fuels (mining trucks can run on electricity, but if solar panels are used to generate it, they would take up an area 2,000 times greater than the trucks themselves). Finally, expanding mining operations can negatively impact biodiversity.
One way of reducing environmental degradation from mining is by instituting environmental regulations. The only environmental regulations currently in place are national ones (some free trade agreements include environmental measures, but the arbitration process is often grueling). Alternatively, environmental degradation from mining can also be reduced by adopting new technologies like air quality control systems on smelting furnaces, recycling waste water and layering tailings paste, or increasing focus on energy management. However, since both regulations and technology tend to be better in wealthy countries, they should collaborate with poor countries to establish sets of best practices. Rich countries should also help create an international protocol coordinating existing national environmental treaties to simplify compliance for miners.
In addition to mining concerns, clean energy technologies leave behind a substantial amount of scrap materials that need to be disposed of at the end of their lifetimes. The waste from solar panels alone could reach 90m metric tons — roughly twice today’s total volume of e-waste — by 2050, and the USA will need to address some 720,000 tons of wind turbine blade material over the next 20 years. Even though burning fossil fuels does not leave behind solid waste like clean energy technologies do, the air pollution emitted by combustion is harmful to the planet and humans. In that sense, it is currently free to pollute and expensive to be green, which creates incentives that lock in high-pollution outcomes.
To fix disposal issues, most commentators suggest moving toward a “circular economy,” but what does that mean in practice? Beginning with product development, products should be designed with minimizing waste in mind. Policies to trigger more of this might look like banning materials which cannot be recycled, investing in research into new materials which can be recycled more, and strengthening extended producer responsibility requirements for products that use critical metals and minerals. While an ideal world would see products that are designed to be recycled actually get recycled, for the time being, secondhand markets can make the economy slightly more circular by extending how long materials get used before being thrown out. However, markets only function well if the incentives make sense. Toward that end, governments should prevent manufacturers from making electrical equipment irrepairable, and investors should seek out ventures hoping to reuse spent parts.
Mineral wealth can also cause social problems within countries. Extraction of some critical minerals today is even more concentrated than oil or natural gas (as shown below), and many resource-rich countries have poor governance and low levels of state capacity. When coupled with economies dominated by natural resources, a condition known as the “resource curse” can arise. Additionally, there are many strategic and geopolitical issues associated with minerals. Historically, OPEC, Russia, and America have been crucial players in maintaining a regular flow of fossil fuels. In the future, geopolitical influence will come from either the possession of raw resources, or innovation in the final technology. Some important questions going forward include whether any advanced economies can move fast enough to challenge China’s current clean energy dominance, whether petrostates like Russia and Saudi Arabia will successfully manage the political and economic reforms to transition away from oil, and whether governments and mining companies can find solutions to eliminate human rights concerns.
When it comes to preventing materials sourced from mines that violate human rights from reaching global markets, transparency is a good start. But what is ultimately needed is a stronger, more unified set of policies agreed to by all countries in the clean energy supply chain, better collaboration between governments and mining companies to provide basic services like education, healthcare, and infrastructure, and the legalization of artisanal mining. In turn, rich countries should place more emphasis on manufacturing than material supply. While clean energy infrastructure does not scale according to Moore’s Law, many integral components do. Thus, the attributes necessary for energy success in the future will be similar to technology more broadly — entrepreneurship, innovation, and dynamism. By rapidly scaling up manufacturing capacity, countries can win influence abroad by leading the world in clean energy innovation and deployment, while creating loads of jobs.
In many ways, the current energy transition represents a tradeoff between one set of environmental and social challenges for another. Yet, it bears reiterating that replacing fossil fuels with carbon-free alternatives will have much lower impacts compared to the status quo over the long run. In order to deploy clean energy technologies as swiftly as possible, investments in mining must keep up with demand growth to keep prices low. In doing so, however, it is important not to lose sight of the concerns surrounding them.
-----
This thought leadership article was originally shared with Energy Central's Energy Collective Group. The communities are a place where professionals in the power industry can share, learn and connect in a collaborative environment. Join the Energy Collective Group today and learn from others who work in the industry.
-----
Earlier:

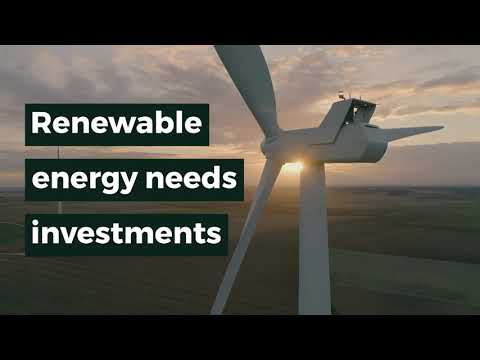
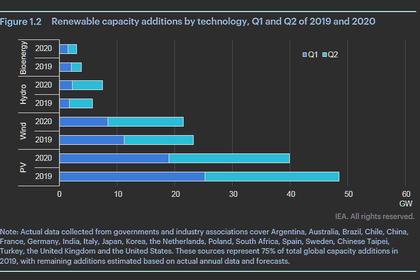
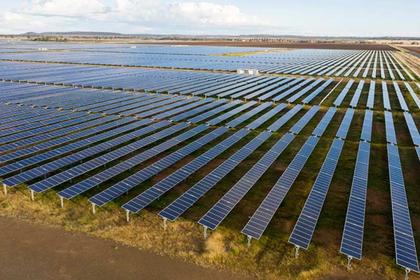
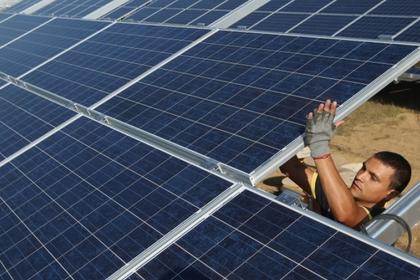
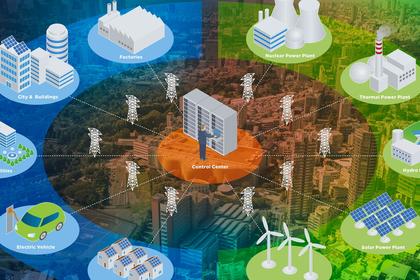