THORIUM FUSION ENERGY
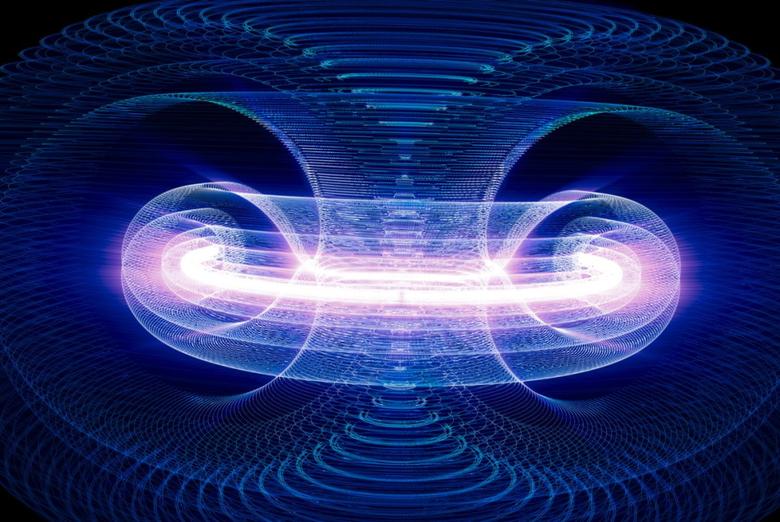
![]() |
|
Current Systems:
Energy production is playing an increasingly important role in the world, and energy demand is growing. The most significant of the different types of energy is the production of electricity. Of course, there are also problems. Such as the tightening of the carbon quota. Fossil fuel power plants are currently in the majority in the world (using coal, lignite, coal, gas, oil).
The renewable energy sources and the share of green energy in energy production is growing such as wind energy, solar energy, biomass, pyrolysis and the resulting gases. The problem with these types of energy is that they are produce locally both in space and time. For example, this means that there is not enough wind energy inside Europe to meets existing needs. In the north and winter, the lack of solar radiation can cause an energy shortage. These systems have to be connected by transmission lines and often require electricity.
Nuclear energy is a way out, but there is actually quite a bit of resistance to it since Fukushima and Chernobyl, although it should be added that both accidents were basically down to design, use, and therefore created by human error or natural phenomenon and not a theoretical problem with the nuclear power plant.
If we look at nuclear energy, there are currently power plants, widespread in the world, operating with U235 the formation of which date back to the 2nd World War.
In practice, we can distinguish between the so-called nuclear methods used for energy production fusion energy and nuclear fission. The production of fusion energy actually takes place, in nature in the Sun and in the stars, and can provide a great deal of energy. Different hydrogen isotopes need to be compressed to overcome the electrostatic repulsion of the nucleus at high temperatures with a very high energy investment. This means that hydrogen isotopes in the plasma state must be compressed at very high temperatures and pressures, which eventually turn into helium nuclei and release the bonding energy due to lack of mass as thermal energy as well as electromagnetic radiation.
I have heard quite a few times in my life that there are only decades left of actual operational fusion energy production. This kind of research is underway around the world and Korea has currently managed to produce the longest duration of fusion plasma at 70 seconds. I think it will take a long time for this to become permanent. The problem is that if the plasma touches the wall of the generator, it simply disintegrates and thus has to hold together with a very strong magnetic field, if anything goes wrong with the stability, the plasma is broken. The other problem is unplugging the energy. We're not there yet to deal with it.
A common feature of the nuclear power plants currently in use is that they all work with nuclear fission. The essence of nuclear fission is that heavy, high-mass metals split into lighter, smaller-mass, and license plate elements as a result of neutron irradiation, while emitting neutrons themselves. The number of these increases from 1 upwards and these neutrons form another fission.
In nuclear power plants, it is very important to maintain balance, so the neutrons generated during the time unit and the subsequent decomposition must be in balance, otherwise the reaction will run, and this is called an atomic bomb.
Ede Teller during World War Two drew attention to the possible fuels of nuclear power plants. Two of these currently seem promising, both of which are chain reaction types. One is U235, which has the problem that natural uranium is only about. 0.72% U235, the rest of uranium is mainly the U238 isotope. However, for a nuclear power plant to operate, 3-4% pure U235 must be produced. They can only produce this from natural uranium with quite complex enrichment. Uranium isotopes of different weights are separated by gas centrifuges. The other problem with uranium is that after the chain reaction runs, the residue is plutonium. In fact, this is why the first nuclear power plants were built, due to the production of plutonium, because the so-called. critical mass is required to produce a nuclear weapon. Plutonium alone would also be a very good energy supplier, as it emits more energy than it needs to use, but 10-100 thousand years should be provided for the storage of fissile products (as long as it shows strong radioactivity), which is too large according to current human scales. Current nuclear power plants are here. In practice, the separation of plutonium from other fissile products is a rather complicated and expensive process, but it is also suitable for energy production and can be produced in breeding reactors - their spread is not yet typical in the world.
Thorium:
There is a very special material that could be used to release significant energy without really leaving radioactive fission products with long decomposition times. This is approx, it only requires 100 years of storage. This element is thorium.
The radioactive decay of thorium is very special. Its half-life is 14 billion years, so it is more than the time of the existence of the universe. Therefore, the natural thorium alone is pure Th232, during which the following nuclear processes take place:
Th232 (1.405 · 1010 years), Ra228 (5.75 years), Ac228 (6.25 hours), Th228 (1.9116 years), Ra224 (3.6319 days), Rn220 (55.6 s), Po216 (0.145 s) Tl208 (3.053 min), 208Pb (stable). Half-lives are displayed in parentheses.
The situation changes when we bombard the thorium with a neutron of adequate energy. It is then excited into the U233 isotope, which releases much more energy than is invested in the absorption of the neutron in the nucleus. Currently, many countries deal with thorium power plants, the most significant of which is India. There is so much thorium treasure that there is a so-called desert of thorium sands. Because thorium alone has a very long half-life, it does not need to be enriched. A significant portion of its isotopes that exist are - almost purely - Th232. Of course, a neutron source is needed to produce energy. Currently, this is solved with a mixed system, which means they mix U235, which provides neutrons. It has reactor moderators that slow down high-speed neutrons to a speed that has an cross-section of effect corresponding to thorium for neutron capture. One problem here is what kind of moderator is used. What has been tried so far consists mainly of moderators suitable for Candu-type heavy water power plants.
They also make the thorium and U235 embedded in graphite balls and this is passed through a reactor space where a mass is already formed that virtually produces neutron radiation at equilibrium. (graphite moderator) Very high temperature, so graphite should not be operated with water (such an explosion was in Chernobyl), but practically cool the reactor with some gas - usually helium gas - and generate steam or heat through a heat exchanger (this part is the same as the classic nuclear power plants).
There is a nuclear power plant where the heat dissipation is medium, the Molten salt, which consists of halogens of various alkali metals, primarily fluoride. The melting point of these is quite low, it does not boil, so enough heat energy can be dissipated with it. There are a smaller number of metal melt systems, which usually dissipate heat with bismuth melt or sodium melt. What is novel and allows the production of thorium nuclear power plants is that they can have very small reactors. These are not feasible with conventional U235.
What is very important for conventional uranium reactors is the critical mass that must be in order for the chain reaction to be continuous. There is no such condition for thorium - almost any small size can be done.
The solution seems to be very practical, not to produce neutron flux with U235 or another neutron-radiating element, but with the so-called. neutron generator. This issue has already arisen in the 1990s. The only problem is that the neutron was produced with a huge linear accelerator at the time, by firing protons at a metal object made of heavy metal at the end of the generator, mostly lead, and neutrons exited from it. This is quite energy intensive and its size determines how much reactor can be made.
The Solution:
The essence of the solution that is proposed is that neutron generators have undergone very great development - a neutron generator of integrated circuit size can now be made. These work on a completely different principle than traditional ones. This is more of a fusion system. It is possible to produce larger neutrons more efficiently with less electricity. Together, the two reactions, i.e. the production of the neutron and the energy extracted from the thorium - the latter is needed to produce more neutrons. It is also very important that this system is completely safe, so the critical mass cannot form because there is no chain reaction.
The spectrum of the neutron generator can be changed by the accelerating voltage as well as the neutron flux. Another important thing is that an on and off reactor can be produced. This technology seems much more advantageous than renewable energy sources, where more and more transmission lines need to be built to connect consumers with energy producers far from them. This means that there is a need for a very long transmission line between Central Europe and Western Europe, which is expensive and means quite a lot of energy loss.
There is no reason why heating plants based on this operating principle, which may be suitable for heating residential buildings or even smaller or larger housing estates, should not be built.
-----
Earlier:
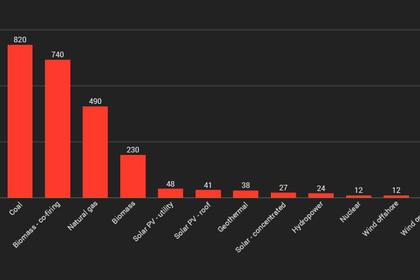
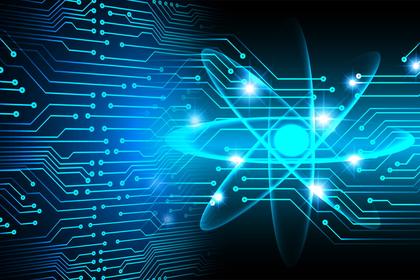
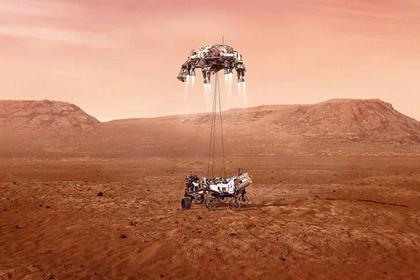
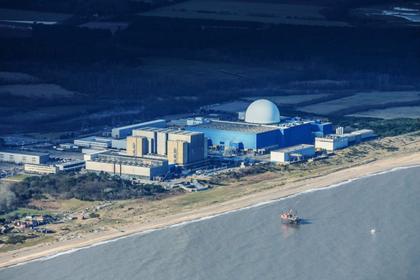
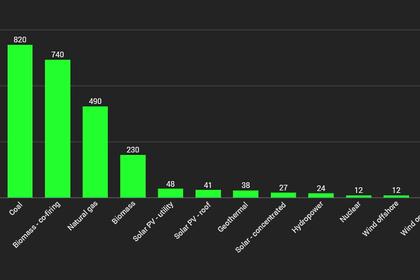
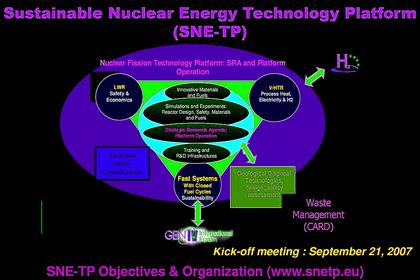
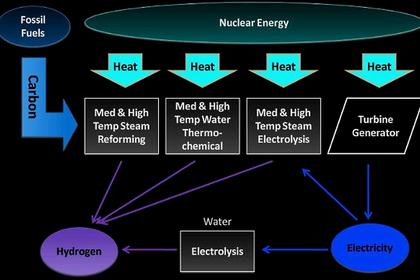