HYDROGEN ECONOMY & CCS
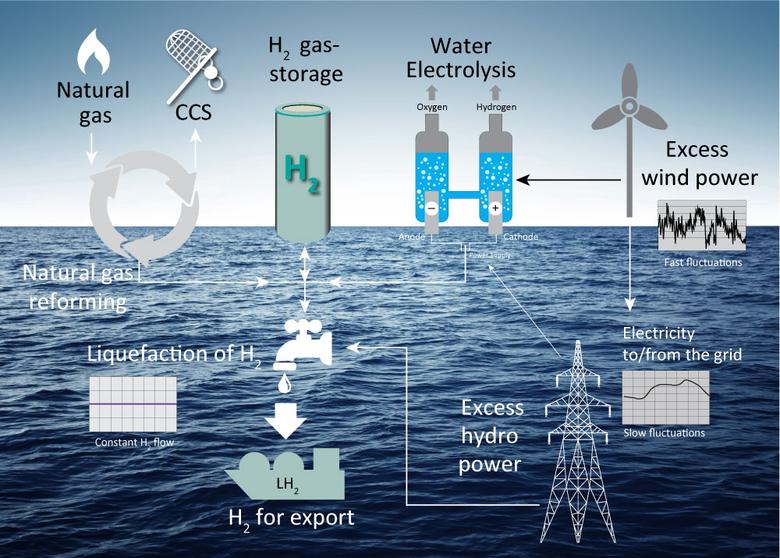
By ROGER ARNOLD Director Silverthorn Institute
ENERGYCENTRAL - May 3, 2021 - I wrote previously about the strategic case for CCS in a hydrogen economy. The focus of that case was on how best to advance the cause of decarbonization in the face of opposition by those whose financial interests are strongly aligned with the status quo. But strategy isn’t defined and executed in a vacuum. It follows from and must account for a range of relevant technical and economic considerations. Here we’ll look at some of those considerations and how they underpin the case for CCS in a decarbonized hydrogen economy.
Energy and cost advantages of blue hydrogen
The gist of the technical case for CCS in a hydrogen economy is that, so long as fossil fuels are available and deliver a high energy return on energy invested (EROEI), it is inherently cheaper and more efficient to produce hydrogen from fossil fuels by reforming, rather than by electrolysis of water. That holds even when the cost of capturing and sequestering the CO2 byproduct of reforming is considered -- provided that the process employed for this “blue” form of hydrogen production is one that has been designed for carbon capture. More about that shortly.
To understand why production of hydrogen from fossil fuels enjoys such an advantage over electrolysis from water, one need only look at the associated chemical reactions and reaction enthalpies. Taking natural gas (methane) as our baseline hydrocarbon, we have:
CH4 + 2H2O ⇒ CO2 + 4H2 ΔH = 27.5 MJ / mol H2
For water electrolysis, we have:
H2O ⇒ H2 + ½O2 ΔH = 241.8 MJ / mol H2
Both reactions are endothermic, meaning that we must supply energy to drive them. But the thermodynamic energy required per unit of hydrogen is 8.8 times more for electrolysis than it is for reforming from methane. To make matters worse, the energy for electrolysis must be electrical, while reforming only requires thermal energy. Electrical energy is the highest “quality” of energy (i.e., has the highest ability to perform work), and is typically valued 3 to 4 times higher than the same amount of thermal energy. (In a heat engine, it typically takes about three megajoules of thermal energy to produce one megajoule of electrical output.) So there’s an order of magnitude difference in the cost of energy for reforming vs. electrolysis. That difference ultimately translates to similar large differences in the specific capital cost of equipment for hydrogen production.
The lower energy and capital cost for production of hydrogen by reforming is of course offset in part by the higher cost of feedstock. For electrolysis, the feedstock is water -- essentially free. For reforming, the preferred feedstock is natural gas. It’s cheap now, but far from free. As gas grows more expensive and renewable electricity grows cheaper, there will eventually be a crossover. “Green” electrolytic hydrogen will become cheaper to produce than blue hydrogen.
Most of the world is still very far from that point. There are only a few exceptions. In countries that have no oil and gas resources of their own, no port facilities or limited port facilities for importing LNG, but ample renewable resources, green hydrogen will already be the better option for hydrogen production. Hence the issue of “blue vs. green” is not a sharp either-or choice. Markets for both do exist, and both will be expanding. But for now and probably for the next two decades in most of the world, blue hydrogen will be far more cost-effective.
Sustainability
Some proponents of green hydrogen argue that since production of hydrogen from fossil fuels is not sustainable in the long run, anything invested in blue hydrogen production will ultimately be wasted. Worse, it will distract from and delay the more important goal of rapid expansion of renewable energy resources.
That argument is flawed at multiple levels. It’s quite true that production of hydrogen from fossil fuels is not sustainable over centuries. The supply of fossil fuels is finite. But it does not follow that investing in production of clean hydrogen from available hydrocarbon fuels will be wasted effort.
Our immediate and urgent problem is to arrest rising levels of CO2 in the atmosphere. Wind and solar resources are a partial means to that end. They are not the end itself. If blue hydrogen can offer a faster and more economical way to achieve the goal of slashing carbon emissions, it is what we should prioritize. Time is critical. It will be at least several decades before depletion of fossil hydrocarbons can render blue hydrogen uneconomical. Long before then, the investments we make will have paid off. The payoff will be in creation of essential infrastructure for hydrogen utilization and power generation -- regardless of the source of hydrogen -- and in reduced levels of CO2 in the atmosphere.
Another important consideration is that even after fossil gas becomes too expensive to compete with renewably produced green hydrogen, it will hardly be “game over” for reforming. There will remain other, fully sustainable feedstocks for production of blue hydrogen. Biogas from anaerobic decomposition of sewage and food wastes, landfill gas and pyrolysis gases from disposal of municipal solid wastes, ethanol from fermentation of crop wastes -- all are prime feedstocks for low energy investment H2 production.
The total amount of such sustainable feedstocks for hydrogen production will be only a fraction of the amount of natural gas now being used for power generation. On the other hand, the latter is far more now than it will need to be in the future. Even if natural gas should continue as our main resource for dispatchable backing power, grid-scale battery storage has advanced to the point that, for short term backing power, battery storage is more economical than gas-fired peaking plants. In the future, it’s conceivable that sustainable feedstocks for blue hydrogen will be sufficient to satisfy nearly all of the power sector’s need for clean hydrogen.
It should also be emphasized that, while green hydrogen production can be carbon neutral, carbon neutrality will ultimately not be enough. It will not stabilize atmospheric CO2 at levels sufficient to achieve the Paris Accord’s “below 2℃” global warming target. Because of the CO2 increase that has already occurred and the inevitable overshoot before even the most optimistic scenarios can get us to zero emissions, the IPCC projects that we will soon have to start removing CO2 from the atmosphere. Producing blue hydrogen from sustainable feedstocks (biomass-derived) may be the most practical option for doing so on an adequate scale. Hence, far from being a dead end, the CCS infrastructure that forms an essential part of blue hydrogen production is something we’ll need anyway for a sustainable climate solution.
When “green” hydrogen isn’t green
There’s an existing market for water electrolysis that Market Watch estimated at $193 million for 2020. But the hydrogen produced doesn’t qualify as green. It’s made by electricity drawn from the grid, and a large fraction of that energy is presently supplied by fossil fuels.
The carbon footprint for electrolytic production of hydrogen, when the electricity is derived from fossil fuels, ranges from 14.8 to 50 kg CO2 / kg H2. The low end is for electricity from a CCGT at 60% thermal efficiency. The high end is for a coal-fired plant at 40% thermal efficiency. By contrast, “gray” hydrogen produced by conventional steam methane reforming (SMR) emits only 7.86 kg CO2 when there is no carbon capture. With conventional CCS, it drops to about 1.7 kg CO2. With a modified reforming process specifically designed for carbon capture, emitted CO2 is under 100 grams. So even gray hydrogen beats grid-powered electrolytic hydrogen by a sound margin on both cost and carbon emissions; blue hydrogen blows it away.
For truly green hydrogen, it isn’t even sufficient for the electrolysis to be powered by renewable energy. The energy used must actually be surplus. It must be energy that, if not used to produce hydrogen, would be wasted. Otherwise, there was some competing demand for the energy. If that was the case, then using the energy to produce hydrogen forced the competing demand to obtain supply from somewhere else. But the only way to obtain more supply when there is no idle RE capacity available is dispatchable generation from fossil fuels. Hence, production of “green” hydrogen from renewable energy that isn’t surplus is not “green” at all. It’s equivalent to producing it from fossil-fueled generation, and increases net carbon emissions.
A fully decarbonized grid can be characterized as one that always has some degree of idle zero-carbon generation capacity in reserve for serving marginal load increases. Hydrogen stored as fuel for dispatchable zero-carbon generation is an attractive way to achieve that. The fastest and most economical way to get there, however, is with blue hydrogen rather than green. Blue hydrogen is a low-energy route to hydrogen production, and begins to pay off immediately in reduced carbon emissions. Green hydrogen, on the other hand, increases total electrical load. There’s a period of increased fossil fuel consumption and increased carbon emissions that must be overcome before the transition begins to pay off.
Premature lock-in
Another point regarding blue vs. green hydrogen is subtle and a bit difficult to explain -- but nonetheless important. Scientists and engineers experienced in startup development of new technologies come to understand it implicitly. However, its importance is hard for those who haven’t experienced it to fully appreciate.
There’s a Christian bible verse that has ironic relevance: Ecclesiastes 9:11.
I returned, and saw under the sun, that the race is not to the swift, nor the battle to the strong, neither yet bread to the wise, nor yet riches to men of understanding, nor yet favour to men of skill; but time and chance happenth to them all. (King James version)
Translated into the mundane realm of technology development and startups, it means that a potentially superior technology and $2.10 will get you a cup of coffee at your local coffee bar.
When it comes to what gains a foothold and flourishes in the market, “time and chance” often have a lot more to do with it than intrinsic technical merit. Being first to achieve market share, often as a result of luck in personal contacts and the vicissitudes of the funding process, can give even a mediocre technology a strong competitive advantage over alternatives. That holds even if the alternatives are potentially superior.
Incumbent technologies can draw on Wright’s law (experience curve) to deliver incremental cost improvements, while competition still struggles with birthing pains. The incumbency advantage isn’t absolute; new, inherently superior technologies do sometimes manage to unseat a prior incumbent. However it takes a strong inherent advantage, and usually the aid of an experienced champion with deep pockets, to pull it off.
As I see it, there’s a very real danger that the rush to embrace green hydrogen will award a premature market lock-in to a technology that isn’t really what we want for the long term. Water electrolysis is just not an energy-efficient way to produce hydrogen. The specific capital cost of electrolysis equipment is high and the round-trip efficiency of P2G2P (Power to hydrogen Gas to Power) for long term energy storage frankly sucks!
Given time, it’s certain that technologies that are inherently more efficient than conventional water electrolysis will arise. But it does take time, and usually a sheltered market environment, for new technologies to succeed. I worry that if we take a path that gives conventional water electrolysis early dominance, more efficient alternatives will be shut out.
We can’t afford to “kick the can down the road” any further when it comes to cutting carbon emissions. Extreme weather events and abrupt climate change are ticking time bombs, and we’ve already wasted too much time in moving to address them. However, if there’s a path that lets us move quickly on cutting carbon emissions while leaving the door open as to the precise nature of a long term sustainable solution, I’m in favor of leaving the door open. Prioritizing blue hydrogen does that.
Addendum: Energy cost of blue hydrogen
Some technically knowledgeable proponents of electrolytic hydrogen will point out that the reaction enthalpies I gave earlier overstate the actual efficiency advantage of reforming. If one is only considering conventional steam methane reforming (SMR), they’re correct.
For simplicity, I gave the SMR reaction as it’s often expressed:
CH4 + 2H2O ⇒ CO2 + 4H2 ΔH = 27.5 MJ / mol H2
That’s thermodynamically correct for the overall reaction. However, conventional SMR -- which is today’s predominant method for making hydrogen from natural gas -- actually takes place in two stages. The first is the SMR reaction proper:
CH4 + H2O ⇒ CO + 3H2 ΔH = 68.7 MJ / mol H2
The second is the water gas shift reaction:
CO + H2O ⇒ CO2 + H2 ΔH = - 41.2 MJ / mol H2
The first reaction is endothermic, while the second is mildly exothermic. Adding the two reaction enthalpies together gives the mildly endothermic 27.5 MJ / mol H2 for the overall reaction. That’s the result I quoted, giving reforming a theoretical 8.8 to one energy advantage over electrolysis. As I said, thermodynamically correct. However it’s not achievable in practice.
In practice, the water gas shift reaction is conducted in a separate reaction vessel, at a lower temperature, and with a different catalyst than the SMR reaction proper. Because of the lower temperature, the heat it gives off cannot be used directly to help drive the first reaction. Some of it can be used to make steam and to preheat the reaction gases, but most of it will be wasted. Hence the effective enthalpy for the overall reforming reaction is closer to 52 MJ / mol of H2 rather than 27.5. That knocks the energy advantage of reforming over electrolyzing from 8.8 to one, down to a bit less than five to one.
A near five to one energy advantage is still quite large. However, if one posits a high energy cost for CCS and adds that into the equation, the energy advantage of reforming begins to look less solidly compelling. With reductions in the capital cost of electrolysis equipment and cheap renewable energy, electrolytic production of H2 might manage to compete with reforming.
The problem with that line of argument is that it pits projected cost reductions in wind and solar energy and electrolyzers against the conventional SMR process as currently implemented. That process, suboptimal for energy efficiency and carbon capture, is nonetheless taken as the way that reforming must work. Yet it was pioneered over a century ago, at a time when CCS was not on anyone’s radar. The model for blue hydrogen that green hydrogen advocates assume simply appends a CCS step to the old process. The assumed CCS step is flue gas scrubbing with an amine sorbent solution. Circulating the solution through a gas-liquid contactor and heating the charged solution to release the captured CO2 is energy intensive.
All of that naturally increases the capital, energy, and financial costs for blue hydrogen. It’s the basis for statements often found in green hydrogen articles about the high cost of blue hydrogen compared to gray. But the model is a strawman.
With incentives to reduce costs and CO2 emissions, there are a number of ways conventional SMR could be improved. One would be with high temperature heat pumping. That would allow all of the thermal energy produced in the downstream water gas shift to be used in driving the initial SMR reaction. The inevitable losses from inefficiencies in the heat pumping process wouldn’t matter. They would appear in the high temperature output of the heat pump, where they would help supply the difference between the enthalpy produced in the shift reaction and that consumed in the initial SMR reaction. The modified SMR process would burn none of its methane feedstock for heat to drive reforming. Reforming would be driven mostly by clean electrical energy supplied to the heat pump.
At least four other avenues for more efficient reforming with carbon capture have been explored. One is Hydrogen Production with Integrated CO Capture from Norwegian firm GTI Energy. Two more are Efficient hydrogen production with CO2 capture using gas switching reforming and Hydrogen Production using Membrane-Assisted Autothermal Reforming Integrated with Chemical Looping Air Separation.
A fourth process is detailed in a Nature Energy paper from 2017, Thermo-electrochemical production of compressed hydrogen from methane with near-zero energy loss. An update was included in a presentation by the European research organization SINTEF at a recent workshop on hydrogen production with CCS. It’s a particularly interesting approach, both in terms of its very high efficiency and its promise of low specific cost and economical operation even at small scale.
The bottom line for all this is that the cost of “blue” hydrogen production in the years ahead will be as much a moving target as the cost of “green” electrolytic hydrogen. Neither approach has a monopoly on technical innovation and cost reductions. With the cost of carbon capture already covered in newer processes for blue hydrogen, the large inherent difference in energy cost will continue to favor blue hydrogen over green.
-----
This thought leadership article was originally shared with Energy Central's Clean Power Community Group. The communities are a place where professionals in the power industry can share, learn and connect in a collaborative environment. Join the Clean Power Community today and learn from others who work in the industry.
-----
Earlier:
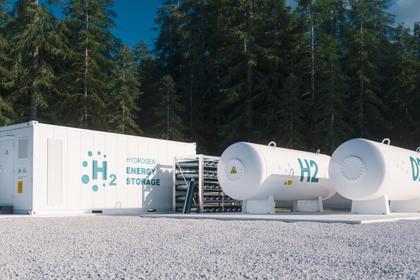
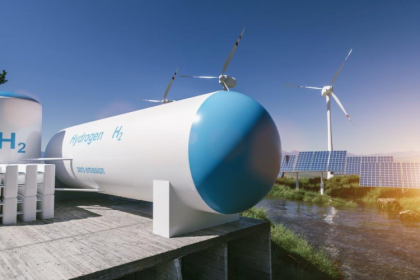
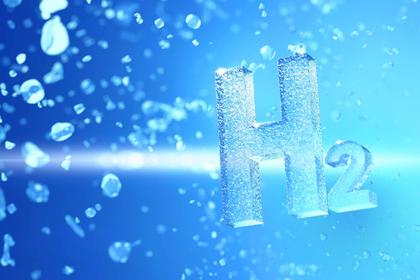
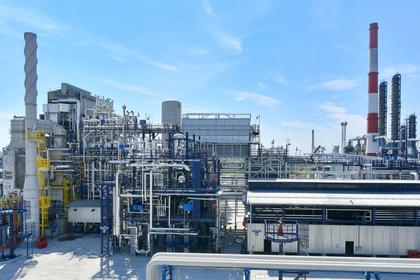
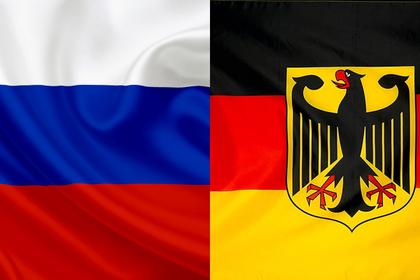
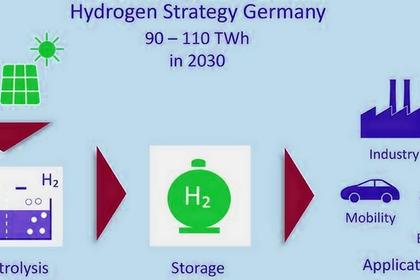
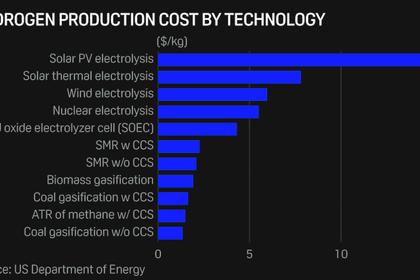